Sign in to get access to all available digital editions of the monthly magazine
Link your Physics World account with your IOPConnect account to get access to all available digital editions of the monthly magazine – you'll only need to complete this step once Company Lanyard
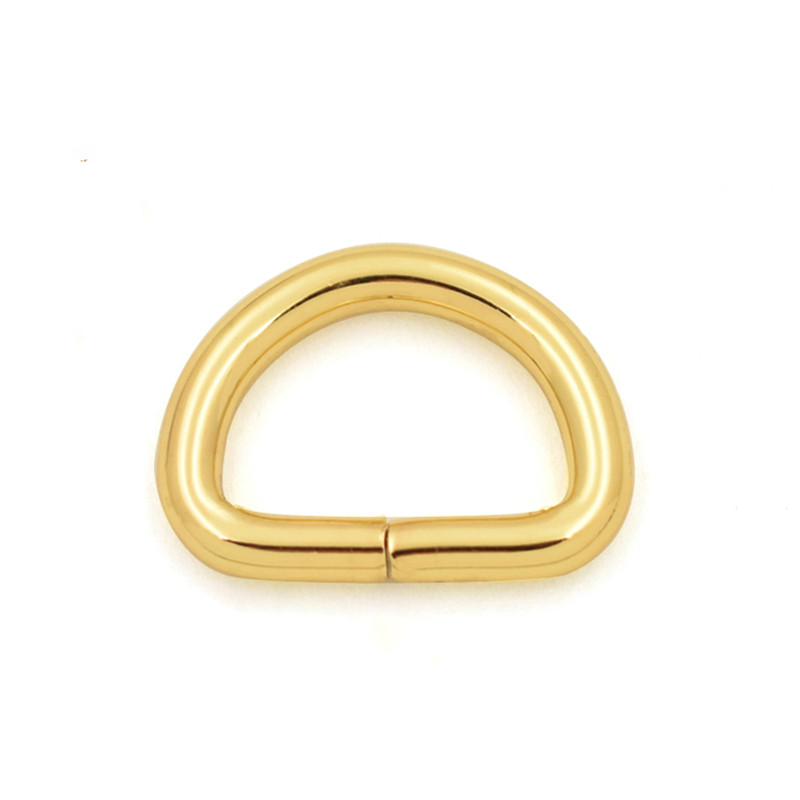
Please enter the e-mail address you used to register to reset your password
Thank you for registering with Physics World If you'd like to change your details at any time, please visit My account
Ribbons of graphene, rather than squares, could make a better platform for probing the unusual electronic effects that arise from twisting and straining adjacent layers of two-dimensional (2D) materials. This is the finding of scientists in the US, Denmark, France and Japan, whose approach differs significantly from previous “twistronics” studies that focused on twisting two flakes of material with respect to each other and then stacking them. According to the team, the new ribbon-based technique could give researchers better control over the twist angle, making the electronic effects easier to study.
In recent years, researchers have found that they can change the electronic properties of 2D materials by stacking layers of these materials atop each other and varying the angle between them. For instance, a bilayer of graphene does not normally have a band gap, but it develops one when placed in contact with another 2D material, hexagonal boron nitride (hBN).
This change occurs because the lattice constant of hBN – a measure of how its atoms are arranged – is nearly the same as that of graphene, but not quite. The slightly mismatched layers of graphene and hBN form a larger structure known as a moiré superlattice, and the interactions between nearby atoms in this superlattice allow a band gap to form. If the layers are then twisted so that they are further misaligned and the angle between them becomes large, the band gap disappears. Similarly, graphene on its own can be tuned from semi-metallic to semiconducting and even superconducting depending on the angle between the individual graphene layers.
To achieve this variety of electronic properties in conventional materials, scientists normally need to change their chemical composition by introducing dopants, or deliberate impurities. Being able to do this in a 2D material simply by altering the twist angle between layers is therefore a fundamentally new direction in device engineering, and has been dubbed “twistronics”.
The problem is that twist angles and the associated strain are hard to control, meaning that different areas of a sample may have inconveniently different electronic properties. In the latest work, a team led by Cory Dean of Columbia University in the US overcame this problem by placing a ribbon-shaped graphene layer (rather than a square flake as is usually the case) atop a layer of hBN and slowly bending one end of the ribbon using a piezo-atomic force microscope. The resulting structure has a twist angle that varies continuously from the point at which the ribbon starts bending all the way through to its end. And instead of uncontrolled variations in strain, the sample now has a uniform strain profile that can be fully predicted by the boundary shape of the bent ribbon.
In their experiments, which are detailed in Science, Dean and colleagues bent one of the graphene layers into a shape that resembles a semi-circular arch. Then they placed this layer on top of a second, unbent, layer. “When placed together in this manner we intentionally introduce an angle gradient along the arc, and a strain gradient across the arc,” Dean explains. “We find that instead of allowing for random fluctuations in the local twist angle or strain, the combined two layers maintain the angle and strain gradients that we impart during the bending process.”
Bending the graphene ribbon is not easy, however. The researchers managed it by first cutting a ribbon from a larger piece of graphene using an atomic force microscopy (AFM)-based process. Next, they fabricated a separate “slider” from a multi-layer, bulk piece of graphite consisting of a round disc fabricated with handles on the outer rim. This slider was then positioned onto one end of the ribbon and pushed across it using the end of an AFM tip. “The slider can be controlled by the AFM tip and removed after the ribbon has been bent into shape,” explains Dean.
A key feature of this process is that the graphene ribbon’ interfacial friction is relatively low when placed on hBN, meaning it can be bent under load, yet high enough to allow the ribbon to hold its bent shape when the load is released.
The extent to which the ribbon will bend depends on the length and width of the ribbon and how much force is applied to the end of it by the AFM tip. The researchers found that long, narrow ribbons (that is, ribbons with a large aspect ratio) are the easiest to bend in a controlled way.
Being able to continuously tune both strain and twist angle will give researchers unprecedented access to the “phase diagram” of twisted angles, Dean tells Physics World. “The electronic band structure of twisted bilayer is extremely sensitive to twist angle, with, for example, the ‘magic angle’ being defined with only one tenth of a degree of 1.1°. Slow and controllable twisting means we can map this dependence in a single device to a precision not previously possible.”
And that is not all: since the role of strain on the magic angle bilayer graphene systems is almost totally unknown experimentally, the new technique provides the first opportunity to measure it in a reproducible way. “Technically, the notion that introducing a strain gradient could help to suppress random twist angle variations was an unexpected surprise for us,” Dean says. “This opens up interesting ideas on how to interplay strain engineering and spatially-controlled angle variations to gain further control over the electronic band structure in twisted layer systems.”
The Columbia team is now mapping the strain-angle phase diagram around the magic angle range in twisted bilayer graphene using a combination of transport- and scan-probe spectroscopy. The researchers are also exploring whether they can apply the technique to other 2D materials systems. In semiconductors, for example, bending could guide and funnel excitons (electron-hole pairs), while in magnetic 2D systems, it might be used to create unusual magnetic textures. “Finally, we are exploring ways to achieve bending through electrostatic or other non-mechanical means,” Dean reveals. “These could allow for in-situ dynamic control of the twist angle in bilayer systems.”
Publishing fundamental and applied research of the highest quality covering all aspects of graphene and related two-dimensional materials

Car Key Lanyards Physics World represents a key part of IOP Publishing's mission to communicate world-class research and innovation to the widest possible audience. The website forms part of the Physics World portfolio, a collection of online, digital and print information services for the global scientific community.